"What are the differences between nuclear fission and nuclear fusion? "
Despite sharing the same surname, nuclear fission and nuclear fusion are not the same thing. So, what do they have in common? And more to the point, how do they differ?
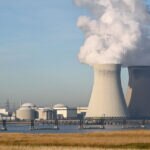
As its name suggests, nuclear power comes from the atom's nucleus, in which the strong nuclear force comes into play, as opposed to electricity, which is generated by the movement of electrons in the outermost shells of atoms on account of the electromagnetic force. Nuclear power arouses passion and hatred among people in fairly equal measure. One of the reasons is that, when talking about nuclear energy without further context, there is no way of telling whether we are talking about fission or fusion.
The study of both forms of generating nuclear energy began in the 1930s, and while nuclear fission energy currently accounts for around 10 percent of the world’s energy, according to the International Energy Agency (IEA), nuclear fusion is still too immature as a technology to be able to generate power on a mass scale. More precisely, since research into nuclear fusion began almost 100 years ago, there have been just two events in which nuclear fusion has released more power than the amount put in to initiate that reaction. On both occasions, it happened at the Lawrence Livermore National Laboratory in Livermore (California – USA), the first time on December 5, 2022, and the second on July 30, 2023.
Nuclear power generation mechanisms
We will now briefly explain the main differences between the two forms of nuclear energy. Although the principle governing these two processes is the same, i.e., electromagnetic repulsion of protons (positively charged particles):
- Nuclear fission occurs when the nucleus of a very heavy atom, such as uranium, which has many neutrons and protons swirling around, splits after colliding with a neutron. The protons of the nucleus, which repel each other because there are a lot of them crammed into a very small space, take advantage of this “nudge” from the external neutron to separate and form two smaller independent nuclei. This process releases energy that can be captured and used to heat water (or other materials), which is then used to drive turbines that generate electricity.
- Nuclear fusion takes place when we manage to overcome the mutual repulsion between two protons and get them to fuse to create a new, heavier nucleus from two separate ones. In this case, the original nuclei are very small (one proton and one or two neutrons), and the resulting nucleus is also fairly small: a helium nucleus with two protons and two neutrons. This process also happens to release energy.
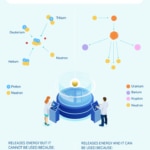
So why does one ‘work’ while the other doesn’t?
Why can this energy be harnessed in one case but not in the other? Because in the case of fission, all we need to do is ‘push’ a neutron to collide with a nucleus, while in the case of fusion, we have to overcome the electromagnetic repulsion between two protons. To overcome this repulsion, a mixture of deuterium and tritium must be heated to around 200 million degrees Celsius (10 times the temperature of the Sun’s core) to obtain a plasma wherein the nuclei and electrons separate and stop forming atoms and begin to move around quickly. A positive magnetic field must also be generated to make the protons join. Both the increase in temperature and the generation of the magnetic field consume vast amounts of energy.
The next big difference between fusion and fission is that, while fission energy is a continuous process that starts when the first nuclei are split and then persists for as long as the neutrons generated under fission continue to smash into the next nuclei, in the case of fusion, the extreme conditions described above are maintained for just a fraction of a second, meaning that the energy generation process needs to be ‘pulsed.’
Many technological challenges must be overcome if we are to unlock the puzzle of nuclear fusion: materials capable of withstanding the hostile conditions found inside the reactors, superconducting magnets that work at low latency and high temperature, the ability to release the internal temperature of the reactor in a matter of seconds to be able to reload it with fuel, building the reactor from materials able to withstand the continuous bombardment by neutrons released during the reaction, and of course being able to absorb the immense amount of energy produced in an instant, among other obstacles.
The waste
Turning to the uncomfortable subject of waste, let’s be clear that both fission and fusion produce radioactive waste, albeit in two different ways.
Nuclear fission generates radioactive waste from spent reactor fuel, i.e., from unstable nuclei that decay, emitting radioactivity in the process but generating so little energy that it cannot be used. Even so, the elements continue to emit radiation that is dangerous to life during the thousands of years it takes for the process to run its entire life. It is little surprise then that these materials must be stored in sealed containers capable of stopping the radiation from escaping.
Nuclear fusion also generates radioactive material since both the inside of the reactor, and the immediate vicinity of the reactor are under constant bombardment from neutrons. Some of these materials wear out with use and, therefore, need to be replaced on a fairly regular basis. This radioactive waste emits radiation but for a shorter time span (hundreds of years), so their storage requires similar levels of security and safety to fission waste, just for fewer years.
So what's next?
According to the International Energy Agency, nuclear fission power generation capacity needs to double by 2050 if we are to meet its Net Zero scenario. This will require further progress in nuclear power generation.
Nuclear fission and fusion technology is evolving, especially regarding the reactors used to generate the power.
In the first case (fission), the new generation of reactors is known as SMRs (Small Modular Reactors). They are being designed to improve safety, make them smaller, improve their external design, reduce costs, toxicity, and the amount of waste produced (by using fuels other than uranium), and improve their performance.
In the case of fusion, the start-ups that are now emerging, fueled by public-private capital, are moving away from the famous “donut” or tokamak (toroidal'naya kamera s magnitnymi katushkami) confinement devices, which was the original design of a nuclear fusion reactor created in 1934, to explore other shapes and technologies. Superconducting magnets at high temperatures, fuel heating by lasers, or microwave oven-like techniques are all part of the new generation of reactors.